Since 1865 GNS Science have demonstrated scientific excellence in a country that straddles two tectonic plates, where earthquakes were first associated with geological faulting, and whose first Nobel Laureate, Ernest Rutherford, saw that radioactive isotopes could be used for geological dating. 'We are proud of our 150-year-old heritage inherited from New Zealand Geological Survey [1865- 1990], DSIR Geophysics Division [1951-1990], Institute of Nuclear Sciences [1959-1992], and DSIR Geology and Geophysics [1990- 1992]. GNS remains New Zealand's leading provider of Earth, geoscience and isotope research and consultancy service. The GNS Science Rock and Soil Mechanics Laboratory is a facility set up for the determination of the mechanical properties of rock and soil materials, based in Avalon, Lower Hutt. The laboratory research team is involved in several research areas ranging from landslide mechanisms in shallow soils to rock mechanics for petroleum geoscience.
Read below or Click here to download the case study.
INTRODUCTION
Large, rapid submarine landslides have the potential to generate tsunami with devastating effects on coastlines and offshore infrastructure. Their failure mechanisms are therefore important but remain to be fully understood. In particular, whilst the role of free gas and gas hydrates in progressive failure has been inferred from geophysical datasets, the physical mechanisms are not well-understood.
The Tuaheni Landslide Complex (TLC) is located on the upper slope of the Hikurangi Margin, off the East Coast of the North Island, New Zealand (Fig 1).
We developed novel laboratory-based testing using gravity core samples from within the TLC and a Dynamic Back-Pressured Shearbox (DBPSB) from GDS Instruments (Fig 2) to replicate offshore slope conditions. The DBPSB was used to test the behavior of the TLC in response to simplified earthquake shaking and increased pore-fluid (PWP) and pore-gas pressure (PGP) within the landslide mass. | 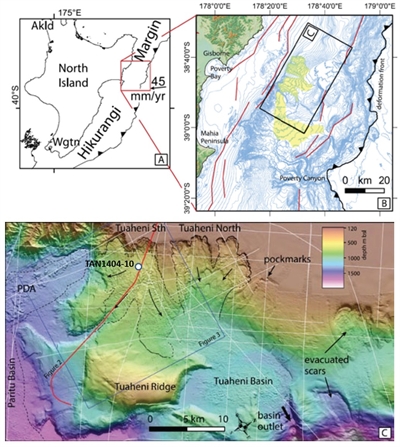
Fig 1. Tuaheni Landslide site and sampling location, (Mountjoy et al. 2014). |
THE SOLUTION
The DBPSB (Fig 2) can simulate effective stress changes at constant shear stress, whilst measuring displacement and pore pressure response. Pore-water and gas pressure increases were simulated by injecting de-aired water and CO² into the sample (Table 1, Fig 3). Simplified earthquake shaking was simulated by applying dynamic shear stresses at a constant normal stress (Table 1, Fig 3).
Horizontal displacement was initiated by elevating pore-water pressure (Fig 4a). Small movements were observed in both tests before the failure envelope was reached (Fig 4b). The rate of movement progressively increased after the envelope had been reached and peaked at the same mean effective stress at both rates of increasing pore-water pressure (Fig 4b). Similar displacement behaviour was initiated by increasing PGP (Fig 4c). Displacement rates during the initial movement are comparable with increasing PWP and initiate close the failure envelope. Rapid acceleration occurred at similar mean effective stress in both samples (Fig 4d).
Dynamic shaking caused very different displacement patterns (Fig 4e). The highest displacement rates were initiated quickly but then diminished with further dynamic shaking without developing excess pore pressure. Shear stresses during DYN5 were not sufficient to reach the failure envelope and therefore minimal, very slow displacements were observed (Fig 4f). Shear stresses during DYN10 did pass the failure envelope and caused more significant displacement during shaking.
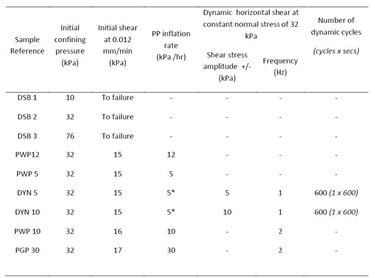
Table 1. Summary lab testing programme. DBS = Drained Shearbox test; PWP = Porewater pressure inflation test; PGP = Pore gas pressure inflation test; DYN = Dynamic stress controlled shear test.*Initial porewater pressure increase to predefined normal effective stress prior to dynamic stage. |

Fig.2 GDS Instruments Dynamic Back-Pressure Shearbox
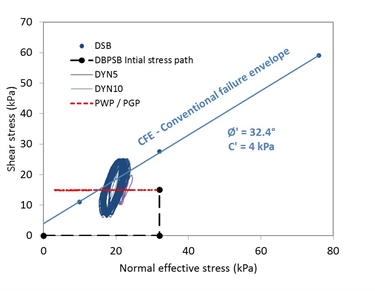
Fig 3. Tuaheni Landslide conventional shearbox and DBPSB testing
CONCLUSIONS AND FURTHER WORK
- The TLC is likely to be susceptible to movement during periods of elevated pore pressure and could undergo significant acceleration.
- Dynamic shaking was shown to contribute less to movement but further testing is required to determine whether this is a credible mechanism to generate landslide acceleration.
- For the first time CO² has been successfully used to
- Testing using N2 is now underway to improve our understanding of gas pressure in landslide movement. (N2 and CH4 have similar density and viscosity, making N2 a good testing analogue).
|
'The GNS Science Rock and Soil Mechanics Laboratory has been working with GDS instruments to develop a range of Geotechnical testing capabilities here in New Zealand. During this time we have found GDS to be and excellent supplier and collaborator. They have shown a very clear understanding of our testing requirements, assisted in the development of novel testing capabilities and have provided high quality |
equipment with a very broad range of potential capabilities. Having purchased equipment from them we have found their staff very quick to respond to our queries both during testing programmes and prior to developing new testing plans. It has been a pleasure to work with such an approachable and professional team and we look forward to developing our relationship further as our research progresses.'
|
REFERENCES
Mountjoy, J. J., Pecher, I., Henrys, S., Crutchley, G., Barnes, P. M., and Plaza-Faverola, A., 2014, Shallow methane hydrate system controls ongoing, downslope sediment transport in a low-velocity active submarine landslide complex. Hikurangi Margin, New Zealand.: Geochem. Geophys. Geosyst., v. 15.
Content for the case study was presented on a poster at the '7th International Symposium on Submarine Mass Movements and Their Consequences, Nov 2015, Wellington, New Zealand'.