What are Gas Hydrates?
Gas hydrates are ice-like substances formed from the combination of methane gas and water under conditions of high pressure and/or low temperature. Such conditions exist under the deep ocean where the gas hydrates may grow within the pore space of a sediment, or as sheets and nodules within it. 1m3 of hydrate contains the equivalent of 164m3 of methane gas at STP (standard temperature and pressure). Dissociation of hydrates, for example due to sea-level temperature rise, can significantly reduce the strength and stiffness of the sediment within which it has formed, and because methane is a powerful greenhouse gas, contribute to global warming.
The abundance of natural gas contained in hydrate and its widespread distribution has led to the potential of hydrate being a vast, as yet untapped, resource of clean, unconventional natural gas. However, the destabilizing behaviour during dissociation indicates that hydrate may also play a role in exacerbating future climate change as well as influence the stability of continental slopes in which it is found.
Recent advances in offshore geophysics have led to the creation of high resolution maps of the sea-floor which have revealed the prevalence of these slides, the largest of which dwarf landslides seen on land, with Pleistocene slides covering tens of thousands of square kilometres, with run out distances of hundreds of kilometres, failures on slopes as low as 0.01º and volumes as large as 20,000km3. The Traenjupet slide off the continental slope of Norway covers an area of 14,000km2, has a volume of 900km3 and a run out distance of 200km. Given the potential devastation these slides can cause both directly and through the generation of Tsunami, there is a need to gain a better understanding of the role of gas hydrate in any of these scenarios, and requires a fundamental understanding of the interaction between the hydrate and the host sediment and how this may change temporally during gas extraction or global warming.
As part of a European research programme (HYDRATECH), a specially designed Resonant Column Apparatus (RCA) was constructed by GDS Instruments for the University of Southampton in the UK, to provide conditions suitable for hydrate growth (low temperature and high pressure). Modifications to the RCA allow both torsional and flexural excitation of specimens so that the shear modulus (G),
Fig 1. Methane burning as synthetic hydrate dissociates at the end of a laboratory stiffness test. |
Young’s modulus (E) and associated damping (D) can be determined on the same specimen. As the resonant column test is non-destructive, tests can be conducted on specimens before, during and after hydrate formation and on specimens where the hydrate has been allowed to dissociate. Methane and argon back pressures can be applied to the specimen, which is tested with nitrogen cell pressure.
Fig 2. Southampton’s RCA within its temperature control system. (Picture courtesy of University of Southampton)
The research showed major differences in stiffness (and therefore strength) of hydrates formed in different sediments. Specifically, tests have shown that the stiffness of sand specimens is markedly increased by the addition of only a small proportion of hydrate within the pore space. Upon dissociation, the pore pressure rises due to the methane being available to the system, which is of course likely to lead to instability in the sediment. See Fig 3 below.
- Grade E Leighton Buzzard sand.
- Confining pressure of 500kPa.
- Hydrated specimens have a target Dr = 0.75.
- At 0% content tests conducted on a sand with Dr = 0.30 and 0.74 respectively.
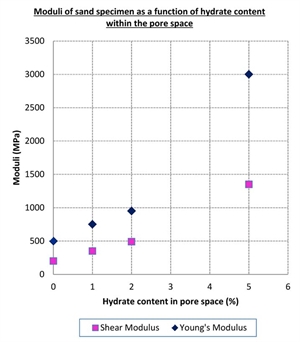 Fig 3. Graph showing moduli of sand specimen as a function of hydrate content within the pore space. (Graph courtesy of University of Southampton) |